Research Spotlight: Drying activities at the LESTE research laboratory (University of Monastir, Tunisia)
Research Spotlight: Drying activities at the LESTE research laboratory (University of Monastir, Tunisia)
At the University of Monastir (Tunisia), we have several active porous-media-related research activities, among which we’ve selected the development of solar dryers to present in this spotlight. As many porous media problems are related to drying of porous materials, we discuss how our economically feasible drying process was modeled, designed, and implemented. This activity is being conducted by the LESTE (Laboratoire d’Etudes des Systèmes Thermiques et Energétiques i.e. Thermal and Energy Systems Laboratory), which resides in the National Engineering School of Monastir of the University of Monastir.
The LESTE was founded in 1999 and currently has 49 permanent researchers, 3 technicians and around 40 PhD students. Twice it has received the prestigious presidential award for scientific research, the first in 2003 and then again in 2020. Research is done collaboratively with other Tunisian and international institutions. The LESTE’s primary research themes are aerodynamics and thermal sciences, including determining material thermal properties, heterogeneous media, combustion and energy system integration, and optimization (www.leste-enim.com).
Design and Optimization of Solar Dryers
Drying food products is one of the oldest preservation methods known to man. It is a high value-added activity, and the dried products are still very popular on the markets of many countries. However, it is an energy-intensive activity. To reduce the energy demand associated with it, one can substitute the conventional fossil fuels (most industrial dryers use natural gas, liquefied petroleum gas or fuel oil) by renewable energy. An appropriate alternative, in countries such as Tunisia, is solar energy.
It is in this context that the LESTE received government funding, with private industry participation, for projects that involve the design, optimization and construction of a solar tomato dryer (funded by the Federated Research Projects program) and a solar wood phytosanitary treatment cell (funded by the National Research and Innovation Program). For both of these projects, numerical models were developed to evaluate alternative designs, select their components and optimize their dimensions. The numerical optimization is based on the operation simulation of the entire solar drying system, including the solar system with an eventual energy storage, the air circuit and the drying cell, over a typical year.
Several technologies and configurations of the solar installation were considered e.g. air and water collectors, flat and evacuated tube collectors, with and without heat storage. After a concept evaluation, utilizing qualitative assessments and rough calculations, the solar dryer configuration of Figure 1 was adopted. In this configuration, hot air passes through the dryer. At its exit the humid (it would have gained moisture extracted from the product) cooler (its temperature drops because of the evaporation) air has to be conditioned before reinjecting it in the dryer cell. This conditioning is obtained by mixing it with ambient air and heating it with the indirect use of solar heated water to adjust respectively the humidity and temperature of the drying air. Prior to its passage through the heat exchanger, the recycled air goes through a mixing chamber where ambient air is admitted to replace the rejected air. The proportions of air rejection are adjusted so that the absolute air humidity has the required value at the dryer inlet. The solar loop consists of vacuum tube solar water collectors, a water circulation pump, a water storage tank and a water-air heat exchanger. A heat storage system is incorporated to ensure the continuous operation of the installation (even when solar energy is not available) and also in order to reduce the collector’s area. In fact, the collector’s area is directly related to the energy requirements. The start of the drying process requires much more energy compared to the remaining periods and without heat storage, the collector’s area would be based on the initial energy requirements. Thus, heat storage allows for a smaller required area, resulting in lower overall investments.
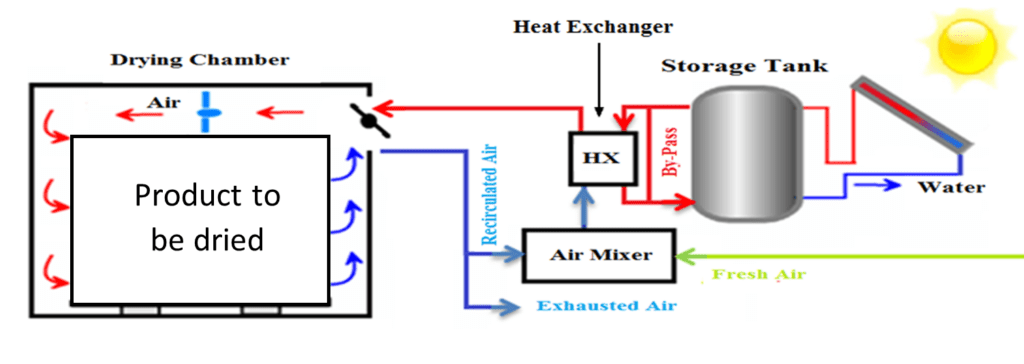
Figure 1. Simplified schematic of the solar dryer installation.
The modeling of both solar dryers is performed in two stages. In the first stage, the air temperature and humidity at the dryer exit are calculated. The second stage is a dynamic macroscopic simulation of the complete dryer, including the solar installation and the air conditioning system, performed with the use of the commercial software TRNSYS ©. The results of this step are used to optimize the solar installation.
In order to conserve energy, as mentioned above, the retained dryer configuration entails the recycling of a large part of the drying air by mixing it with the right proportion of ambient air to adjust its humidity. For this, the temperature and the relative humidity of the air at the outlet of the drying chamber are needed. Interestingly, the calculation methods for the outlet relative humidity is different for tomatoes and wood dryers.
In fact, for the tomato dryer, an experimentally obtained drying kinetics i.e. the tomato water content as a function of time, for given drying air properties, was used along with the first law of thermodynamics, the law of the conservation of mass of water and the psychrometric chart [1]. As this work is part of a federated research program involving several laboratories, the drying kinetics were obtained experimentally by our partners of the project. For the phytosanitary treatment cell, however, the air temperature and humidity at the dryer exit are calculated by an in-house developed finite-difference code based on a detailed 2D modeling of the drying in the wood stack [2].
In order to adopt the most appropriate configuration and subsequently size its components, a critical analysis of several alternative designs was performed. We looked at systems with air and water solar collectors, flat and evacuated tube type, and systems with and without heat storage of different technologies. The result of this iterative process was that the most suitable and affordable configuration that best met the drying needs of both solar dryers was an evacuated tube type water solar collector and a two-level stratified storage tank.
To minimize the initial investment while still ensuring the widest coverage of drying needs of both tomatoes and wood, we optimized the solar system. The adopted procedure is as follows. TRNSYS simulations are conducted, for a typical day of every month of the year, in which the dimensions of the dryer components are adjusted iteratively until the obtained configuration provides the required air flow rate at the desired temperature throughout the entire drying process. Consequently, the required evacuated tube collector area and the volume of water thermal storage are obtained. Figure 2 shows examples of required solar collector areas for tomato dryers (with a capacity of 315 kg of fresh tomatoes) and wood phytosanitary cells (for the treatment of 0.7 m3 of wood) installed in the vicinity of Tunis. The curves of the storage tank volume follow similar variations with a nearly constant ratio of tank volume to collector area of 80 l/m2.
(a)
(b)
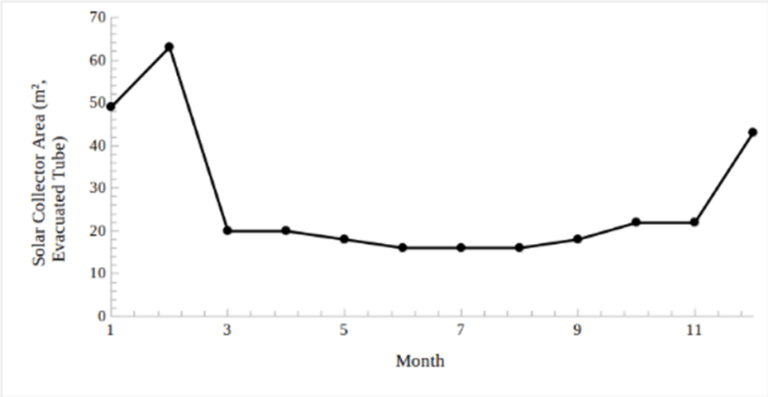
Figure 2. Required collector area variation through the year for (a) the tomato dryer, and (b) the phytosanitary treatment cell.
The needed collector area and tank volume are very high in winter compared to other seasons. Investing in collectors and a tank that would satisfy the winter needs would result in more than doubling the cost for marginal gains. So instead, it is proposed to size the collectors and tank to cover the needs of the rest of the seasons and to reduce the quantity of dried tomatoes or treated wood in winter to the quantity that can be handled by a system equipped with these collectors and tank. To help in the decision making, a rough techno-economic study, based on 2017 prices in Tunisia, was performed to select the appropriate solar collector area and whether to use auxiliary heating by burning Liquefied Petroleum Gas (LPG) or not. Detailed results can be found in [1] and [2].
The constructed prototypes for the tomato solar dryer are presented in the video below, and Figure 3 provides images of the wood phytosanitary solar treatment cell.
Click here to see the video of The prototype tomato dryer.
(a) | (b) |
![]() |
|
(a) | (b) |
![]() | ![]() |
(a) | (b) |
![]() | ![]() |
Figure 3. The phytosanitary treatment cell prototype installation; (a) The solar field; (b) The storage tank; (c) The expansion vessel ; (d) The air-handling unit; (e) The phytosanitary treatment cell; and (f) the cell interior showing the wood stack to be treated.
[1] A. Zriba, M.S. Guellouz and A. Jemni, 2021, Design and optimization of a tomato drying solar cell, Journal of the Brazilian Society of Mechanical Sciences and Engineering, 43:296 https://doi.org/10.1007/s40430-021-03010-8
[2] A. Zriba, M. M. Oueslati, M. S. Guellouz, C. Bouden, A. Jemni, 2022, Design, Optimization and Implementation of a Solar Cell for Wood Phytosanitary treatment (Accepted), In press Energy Sources, Part A: Recovery, Utilization, and Environmental Effects.